Development of biological oxygen probe
- Intracellular oxygen partial pressure measurement and imaging
- Tissue oxygen partial pressure measurement and imaging
- Development of Ratio Oxygen Probe
- Intracellular oxygen consumption rate measurement
The video introduces our research. Please click here to watch it.
Our body is said to be composed of approximately 60 trillion cells. This vast number of cells cannot perform their functions without a constant supply of oxygen and nutrients through the bloodstream. When a human being is unable to breathe for any reason, life is immediately taken from him or her because the oxygen supply is cut off and the cells are unable to function. Therefore, it is very important to detect oxygen in the living body in real time.
In our laboratory, we use “phosphorescence” to detect oxygen. In general, molecular luminescence is divided into fluorescence and phosphorescence. Fluorescence is emission from the excited singlet state, while phosphorescence is emission from the excited triplet state. Phosphorescence has a longer emission lifetime than fluorescence and is characterized by quenching when oxygen is present in the surroundings, resulting in a significant decrease in luminescence intensity and emission lifetime.
Very few substances show phosphorescence at room temperature and are mainly limited to organometallic complexes. Among them, iridium complexes (Ir complexes) are known as substances that show strong phosphorescence at room temperature. When this phosphorescence is used for electroluminescence (OLED), the luminous efficiency is higher than that of fluorescence, and Ir complexes are attracting attention as light-emitting materials for OLED.
We are developing bio-oxygen probes based on Ir complexes as a new application of Ir complexes, which show various luminescent colors depending on the aromatic ligands attached to the Ir ions. The water solubility and cell affinity of Ir complexes can be controlled by introducing hydrophilic or hydrophobic groups to the ligands. Taking advantage of these features, we are designing and synthesizing probes for oxygen sensing and imaging inside and outside of cells. We are also testing whether the synthesized oxygen probes fulfill their intended functions using cultured cells and small animals. Thus, we aim to develop highly practical oxygen probes that can be easily used by biological, medical, and pharmaceutical researchers by conducting molecular design and synthesis, photochemical characterization, and cell and small animal experiments in our laboratory.
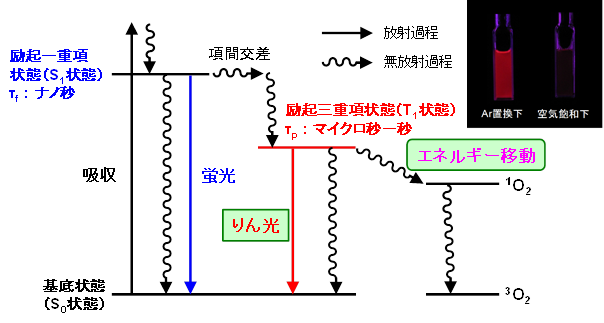

Intracellular oxygen partial pressure measurement and imaging
To reveal intracellular oxygen levels, it is necessary for oxygen probes to be efficiently taken up into cells. We have developed an Ir complex (BTPDM1, Fig. A) that is efficiently taken up into cells by using a ligand with a dimethylamino group, and measured the partial pressure of oxygen in cultured cells.
Figure B shows cultured cells attached to the bottom of a petri dish containing culture medium and living while receiving oxygen supply from the outside world. If this two-dimensional cell layer is covered with a thin glass plate (cover slip), the cells underneath are expected to be in a hypoxic state because the oxygen supply from the top surface is cut off. In the image (Figure C) taken by magnifying the edge of the glass plate with a fluorescence microscope after the cells have previously taken up BTPDM1, the cells inside the plate are more intensely illuminated than the cells outside the plate. Furthermore, when the luminescence lifetime of BTPDM1 was measured for cells inside and outside the plate, the results are shown in Figure D, indicating that cells deprived of oxygen supply have a longer lifetime, i.e., they are hypoxic. By analyzing the lifetime values, the oxygen partial pressure of the cells inside the plate was 6.9 mmHg, while outside the plate was 166 mmHg, indicating that the inside of the plate was considerably hypoxic.
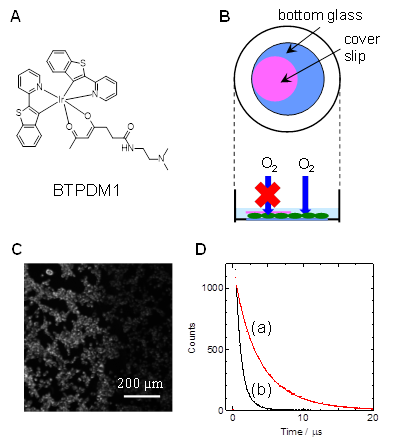
Additionally, we have also investigated the oxygen partial pressure near the edges. Figure A shows a phosphorescence lifetime imaging image constructed from time-resolved luminescence microscopy images. The edge of the cover glass is in the center of the image, and the cells in the left region are covered by the cover glass. Plotting the phosphorescence lifetime along the white line in the image shows that the phosphorescence lifetime of cells not covered by the cover glass is about 1 μs, whereas the phosphorescence lifetime increases inward from the edge to about 4 μs at about 400 μm (Figure B). Converting this to an oxygen partial pressure imaging image shows that there is an oxygen partial pressure gradient over about 400 μm, with oxygen partial pressures inside and outside the cover glass of about 120 mmHg and less than 5 mmHg, respectively (Figures C and D). This is because oxygen diffusing from the sides is gradually consumed from cells near the edges inward. This corresponds to the fact that in tissues, cells in the vicinity of capillaries have sufficient oxygen, whereas the amount of available oxygen gradually decreases as one moves farther from the vessels, making this a good model experiment for considering oxygen partial pressure in tissues.
Currently, we are working on the development of Ir complexes with higher functionality than BTPDM1 and the measurement of intracellular oxygen levels using a microplate reader. Furthermore, we are also conducting research on oxygen diffusion in membranes using Ir complexes.
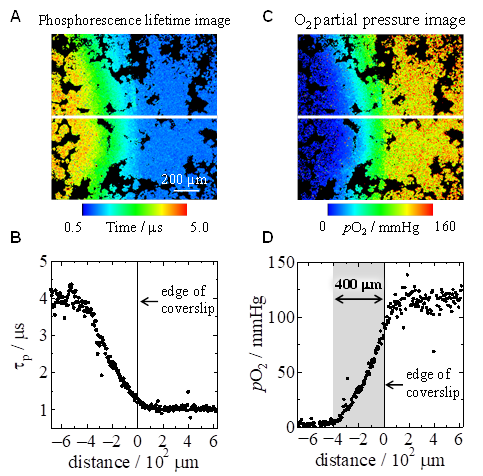
Tissue oxygen partial pressure measurement and imaging
The partial pressure of oxygen in tissues is maintained at homeostasis by the supply of oxygen through blood vessels and the consumption of oxygen within cells. Since the partial pressure of oxygen in the blood decreases from arteries to veins, the amount of oxygen available to cells near arteries is higher, and as a result, the partial pressure of oxygen in cells differs even within the same tissue. The oxygen partial pressure also varies depending on the metabolic activity of the tissue. Furthermore, in hypoxic tissues such as cancer, homeostasis is disrupted, making the situation more complex. Quantification and imaging of such heterogeneous oxygen partial pressure is much more difficult and challenging than in cultured cells. We have been working on oxygen partial pressure measurement and imaging of mouse tissues using Ir complexes as oxygen probes based on their phosphorescence lifetime imaging measurements. So far, we have investigated oxygen partial pressure in kidney, liver, pancreas, fundus, and bone marrow, including collaborations.
The liver is the largest organ in the human body and is responsible for many functions, including metabolism and storage of carbohydrates, fats, and proteins, decomposition of waste products, detoxification of toxic substances, and synthesis of bile for digestion. The liver consists of a basic unit called the hepatic lobule, which has a hexagonal columnar structure (Figure A). At the apex of the hexagonal column is the portal vein (PV) region, which consists of the hepatic artery, portal vein, and bile ducts, and the central vein (CV) is located in the center. Blood flows from the PV region through the sinusoidal vessels to the CV. Oxygen in the blood is supplied to the hepatocytes adjacent to the sinusoidal vessels and thus gradually decreases from the PV region to the CV region. It has been pointed out that this causes an oxygen partial pressure gradient to appear and changes the function of hepatocytes. Therefore, imaging the oxygen partial pressure gradient is important in elucidating metabolic processes in hepatocytes. Figure B shows a phosphorescence lifetime imaging image near the liver surface measured approximately 20 minutes after tail vein administration of BTPDM1 to anesthetized mice. A region with a phosphorescence lifetime of approximately 2.5 μs (PV region) surrounds a region with a phosphorescence lifetime of approximately 3.1 μs (CV region), showing imaging of the liver lobules. The gradual increase in phosphorescence lifetime from the PV region to the CV region indicates the presence of an oxygen partial pressure gradient.
Currently, we are working on oxygen partial pressure measurement and imaging not only in normal tissues but also in pathological tissues such as cancer.
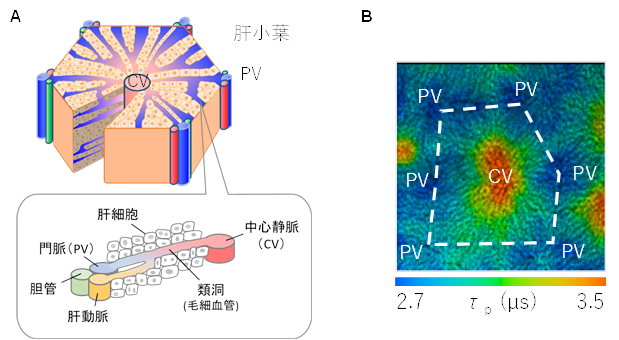
Development of Ratio Oxygen Probe
To determine the partial pressure of oxygen in a cell or tissue using a luminescence probe, the luminescence lifetime must be measured. Generally, the luminescence lifetime is obtained by photoexcitation of Ir complexes in cells and tissues with pulsed light such as laser and measuring the decay of Ir complex luminescence. This requires a special microscope. We designed and synthesized a ratio-type oxygen probe (Figure A) as a simple method to measure oxygen partial pressure without such special equipment. This molecule consists of a fluorescent cluster called coumarin 343 and an iridium complex, BTP, which is a phosphorescent cluster, linked by tetraproline as a linker. Here, the intensity of fluorescence is almost independent of oxygen partial pressure, but phosphorescence weakens with increasing oxygen partial pressure, so the oxygen concentration around the probe molecule can be determined by measuring the intensity ratio of fluorescence to phosphorescence. Figure B shows how the emission spectrum changes with the oxygen partial pressure of the solution, and Figure C shows how the emission color of a solution containing a ratio-type oxygen probe changes with oxygen partial pressure.
We are currently designing and synthesizing linkers to increase cell affinity and developing probe molecules that many researchers can measure with their own fluorescence microscopes.
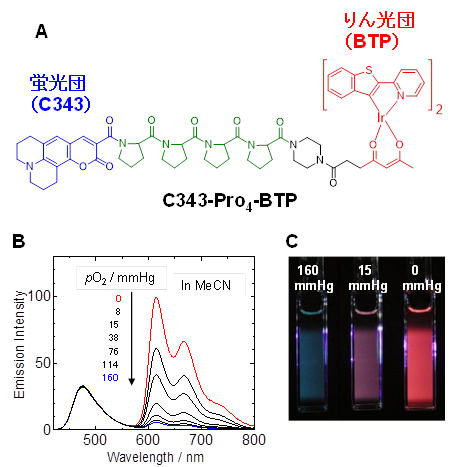
Intracellular oxygen consumption rate measurement
Intracellular oxygen is consumed primarily for ATP synthesis as the final electron acceptor in the mitochondrial electron transfer system. Therefore, changes in intracellular oxygen levels, especially a decrease in oxygen levels, have a significant impact on vital activities. When intracellular oxygen levels decrease for some reason, hypoxia-inducible factor (HIF-1α), which is proteolytically degraded under normal oxygen, becomes stable, and stabilized HIF-1α is known to migrate into the nucleus and increase the expression of various genes to resolve hypoxia. For example, in cells under hypoxic conditions, such as in cancer tissue, the amount of ATP that can be produced by the transition from aerobic to anaerobic metabolism is greatly reduced due to decreased oxygen levels. This leads to increased expression of glucose transporter1 , which increases glucose uptake, and expression of vascular endothelial growth factor, which promotes unregulated angiogenesis. Thus, cells are equipped to respond rapidly to changes in oxygen levels. On the other hand, at what level of oxygen does such a system function? This question requires accurate measurement of intracellular and extracellular oxygen levels and oxygen consumption rate (OCR).
We have identified a methodology to measure OCR in a simple manner by measuring changes in phosphorescence intensity of water-soluble Ir complexes in a microplate reader: cells derived from human colon adenocarcinoma were seeded in 96-well plates, incubated for 48 hours, replaced with medium containing Ir complexes, and sealed with mineral oil in the wells, Phosphorescence intensity measurements were performed on wells to which the deconjugating agent FCCP was added immediately before sealing. As shown in Figure A, the phosphorescence intensity increased with time, and the rate of increase was greater in the wells to which FCCP was added than in the wells to which it was not added. This is because the oxygen concentration in the culture medium decreased due to oxygen consumption by the cells, and the rate of decrease was faster with the addition of FCCP. From the obtained slope of the time variation of oxygen concentration (Figure B) at the initial (0-60 min), the OCR per well with and without FCCP was determined to be 30 pmol min-1 and 129 pmol min-1, respectively. Since the number of cells in each well is almost the same, the addition of FCCP significantly increases the OCR of the cells. A reagent kit utilizing this technique is now in practical use.
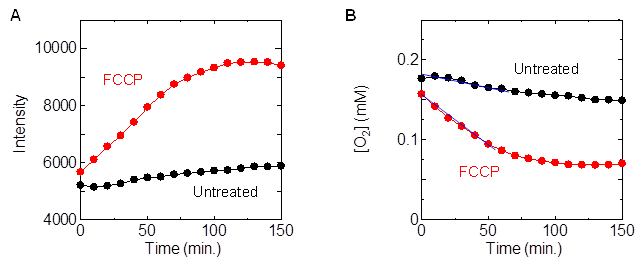
Development of small molecule fluorescent and phosphorescent probes for bioimaging
- Development of lipid droplet (fat droplet) imaging probe
- Development of organelle-selective fluorescent and phosphorescent probes
- Development of Vascular Imaging Probes
- Development of new imaging probes
Life is sustained by cells as the basic unit of vital activity. Cells are partitioned from the outside world by the cell membrane, and a special “reaction field” is formed inside the cell for various chemical reactions to proceed efficiently. In addition to blood vessels and interstitium, tissues also form a “microenvironmental field” due to the grouping of various cells. Since disruption of the homeostasis of intracellular reaction fields or tissue microenvironmental fields directly leads to a crisis of life activity, quantification of various physical quantities (viscosity, pH, temperature, polarity, etc.) and chemical species (lipids, ions, O2, NO, reactive oxygen species, etc.) to understand the temporal and spatial dynamics of these reaction fields and microenvironmental fields is being promoted worldwide. However, cells and tissues are often subjected to However, because cells and tissues are a foreign environment, it is still difficult to quantify targeted physical quantities and chemical species using existing methods that have been conducted in ideal dilute solution systems, and breakthroughs are needed.
Our laboratory aims to create quantitative imaging techniques for intracellular reaction fields and tissue microenvironmental fields in foreign environments from a photochemical approach, and to create breakthroughs that will contribute not only to an integrated understanding of biological phenomena but also to drug discovery, diagnosis, and treatment. Specifically, we are developing small molecule sensors using fluorescence and phosphorescence of molecules and establishing a methodology to quantify physical quantities and chemical species in a foreign environment by measuring the luminescence of these sensor molecules.
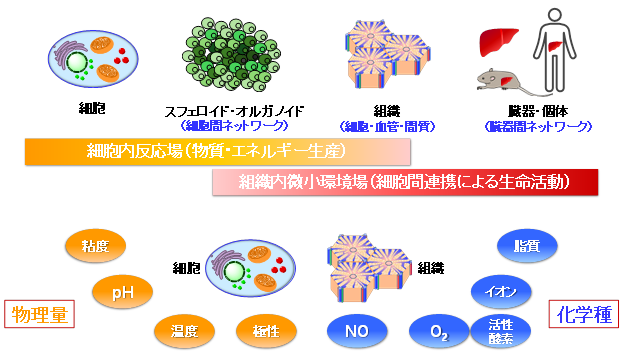
Development of lipid droplet (fat droplet) imaging probe
Lipid droplets (fat droplets) are intracellular organelles that are ubiquitously present in eukaryotic cells and consist of neutral lipids such as triacylglycerols and cholesterol esters surrounded by a phospholipid monolayer membrane that contains membrane proteins. Until recently, lipid droplets have been considered as simple storage organs for excess lipids, but with the recent discovery of proteins and enzymes related to lipid metabolism, they are now recognized as organelles that function dynamically within the cell. In addition, excessive lipid accumulation and metabolic abnormalities at the individual level are associated with diseases such as obesity, diabetes, and atherosclerosis. In particular, it is estimated that more than 10 million people in Japan suffer from nonalcoholic fatty liver (NAFL, NASH: nonalcoholic steatohepatitis), which is caused by excessive accumulation of neutral lipids in the liver. In addition, NASH can progress to cirrhosis or liver cancer if left untreated, so early detection in the fatty liver stage is considered important. For this reason, research on measuring the distribution and amount of lipid droplets in cells and tissues, as well as imaging their formation and disappearance processes, is being actively conducted.
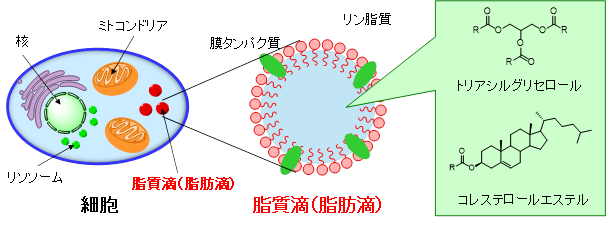
We have developed probes (DBC30, PC6S) that can image lipid droplets in tissue as well as in cultured cells (Figure A). By adding these probes to the culture medium, we have shown that we can selectively visualize lipid droplets in cells (Figure B). We also succeeded in imaging lipid droplets in the liver and enlarged fat droplets in fatty liver by administering them to healthy mice under anesthesia and to fatty liver model mice (Figure C).
Currently, DBC30 and PC6S are commercially available through reagent companies. New fluorescent lipid droplet imaging reagents are also under development, which are expected to contribute to the discovery, diagnosis, and treatment of NAFL and NASH.
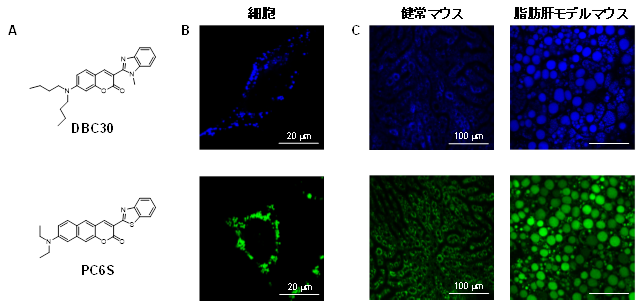
Development of organelle-selective fluorescent and phosphorescent probes
There are a wide variety of organelles in the cell, including the nucleus, mitochondria, endoplasmic reticulum, and lysosomes. By imaging the intracellular distribution of these organelles in real time, a great deal of intracellular information can be obtained. It is also important to image multiple organelles simultaneously because each organelle is linked to each other.
We are developing molecules that can selectively image targeted organelles by binding organelle-selective units to fluorescent and phosphorescent molecules. We are also developing probe molecules with different emission colors to perform multi-color imaging. Furthermore, we are aiming to develop probe molecules that function not only in organelles in cultured cells but also in vivo.
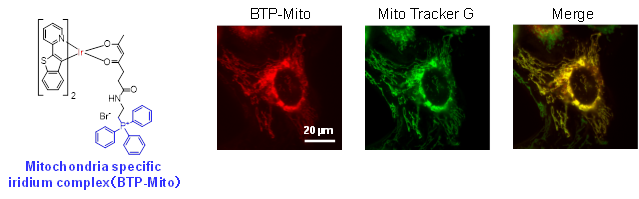
Development of Vascular Imaging Probes
Blood vessels are vital tissues that supply nutrients and oxygen to tissue cells through blood and collect waste products and carbon dioxide. For this reason, abnormalities in the vascular system can directly jeopardize vital activities. In particular, narrowing or tortuosity of blood vessels prevents adequate oxygen supply to the tissues, leading to tissue hypoxia. Such hypoxia is a common feature of cancer.
We found that Ir complexes with oligoarginine peptide ligands (Figure A) bind to vascular endothelium, and successfully used them to image blood vessels in various normal and tumor tissues (Figure B). Since the luminescence of Ir complexes is phosphorescent, it is possible to eliminate tissue autofluorescence and image only blood vessels by using the time-gating method. Furthermore, when coadministered with a fluorescent lipid droplet imaging reagent (PC6S) developed in our laboratory, the narrowing and meandering of blood vessels due to enlarged lipid droplets were imaged in fatty liver.
In addition to the vascular endothelial type, we are currently developing imaging reagents for the blood circulation type.
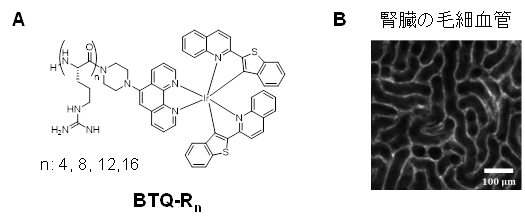
Development of new imaging probes
There are many physical quantities and chemical species targeted by bioimaging. To elucidate them, we need molecules that can sense and image only the targeted physical quantity or chemical species, and each one is a new research topic. In our laboratory, new small molecule fluorescent or phosphorescent probes are designed by photochemical approaches and synthesized by organic chemical methods. We also investigate the properties of the synthesized molecules and improve them for practical use.